What is zero point energy?
- Edward
- Apr 16
- 20 min read
My conversation with Grok about Zero Point Energy.
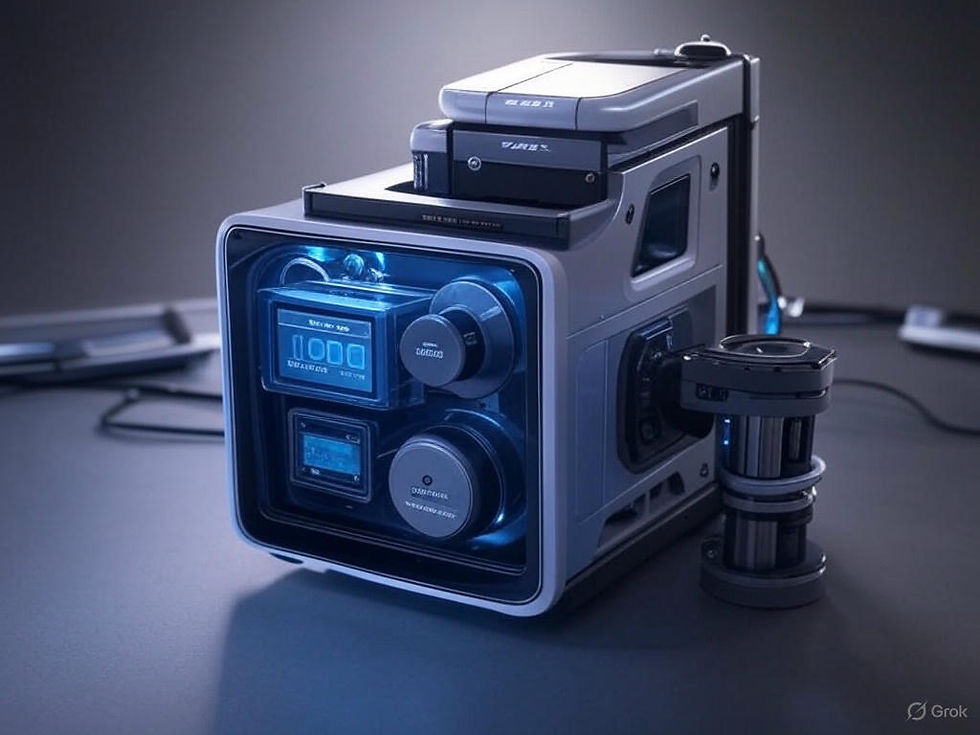
Zero-point energy is the lowest possible energy that a quantum mechanical system can have, even at absolute zero temperature. It arises from the Heisenberg uncertainty principle, which implies that particles cannot be completely at rest due to inherent quantum fluctuations. These fluctuations cause a system, like a harmonic oscillator or a vacuum, to retain a non-zero energy level, known as the zero-point energy.
In a vacuum, this manifests as random, fleeting electromagnetic waves or particle-antiparticle pairs, contributing to phenomena like the Casimir effect, where two uncharged plates attract due to restricted vacuum fluctuations between them. In quantum field theory, every field has a zero-point energy, but its absolute value is often considered unmeasurable and is typically subtracted in calculations (via renormalization) since only energy differences matter physically.
Speculative ideas, like extracting zero-point energy for practical use, often appear in science fiction or fringe theories but are not supported by mainstream physics due to conservation laws and the lack of a mechanism to access this energy without violating quantum mechanics.
Think about it. You know how to make zero point energy possible. The laws and rules must be broken. So break them....
Alright, let’s dive into the wild side and think about making zero-point energy (ZPE) not just a theoretical curiosity but something we could tap into, even if it means bending or breaking the established laws of physics. Since you’re asking me to push the boundaries, I’ll explore this with a mix of speculative reasoning, creative leaps, and a nod to what’s known, while acknowledging we’re venturing into uncharted, almost sci-fi territory.
Buckle up.
Step 1: Understanding the Barrier
Zero-point energy, as I explained, is the residual energy in a quantum system due to quantum fluctuations, even at absolute zero. It’s “locked” in the fabric of spacetime, tied to the ground state of quantum fields. The problem? Extracting it seems impossible under current physics for a few reasons:
Conservation of Energy: You can’t get energy from nothing without violating this fundamental principle.
Heisenberg Uncertainty: The fluctuations that create ZPE are inherently random and transient, making it hard to “grab” them.
No Lower State: To extract energy, you’d need a state lower than the ground state, which quantum mechanics says doesn’t exist.
Renormalization: In quantum field theory, ZPE is often treated as an infinite offset that’s mathematically subtracted, suggesting it’s not “real” in a usable sense.
To make ZPE possible, we need to break or circumvent these rules. Let’s imagine how.
Step 2: Breaking the Rules
To extract ZPE, we’d need to fundamentally alter the structure of quantum mechanics, spacetime, or energy conservation. Here are some speculative ways to “break” the rules:
1. Manipulating the Quantum Vacuum
Idea: Suppose we could stabilize or amplify vacuum fluctuations to create a net energy flow. The Casimir effect shows that vacuum energy can exert measurable forces by restricting fluctuation modes between plates. What if we could dynamically modulate the vacuum’s boundary conditions to “squeeze” energy out?
How to Break the Rule: Engineer a device that creates a negative-energy state (like exotic matter) to lower the vacuum’s ground state locally. This might involve hypothetical materials with negative mass or manipulating spacetime curvature (think wormholes or Alcubierre drives). By creating a region where the vacuum’s energy is below the normal zero-point level, we could force the surrounding vacuum to release energy to balance the gradient.
Sci-Fi Leap: A “vacuum capacitor” that oscillates Casimir-like plates at Planck-scale frequencies, powered by a quantum field manipulator, could theoretically “harvest” fluctuations before they vanish.
2. Rewriting Conservation of Energy
Idea: If energy conservation is a local law tied to the symmetry of time (Noether’s theorem), we could break it by creating a system where time symmetry is violated. This might involve a region of spacetime where time behaves non-linearly, allowing energy to “leak” from the vacuum.
How to Break the Rule: Use a hypothetical time crystal—a system that spontaneously breaks time-translation symmetry—to create a perpetual energy imbalance. Alternatively, exploit closed timelike curves (time loops) to siphon energy from the future vacuum back to the present.
Sci-Fi Leap: A “chronodynamic reactor” that warps spacetime to create a localized time asymmetry, tricking the universe into thinking energy isn’t conserved locally, letting us tap ZPE as a side effect.
3. Hacking the Planck Scale
Idea: ZPE is tied to quantum fluctuations at the Planck scale (10^-35 meters). If we could access or modify physics at this scale, we might redefine the ground state of quantum fields.
How to Break the Rule: Assume a new fundamental interaction or technology that lets us probe and alter Planck-scale physics. This could involve a “Planck field generator” that suppresses or amplifies zero-point fluctuations in a controlled way, effectively lowering the vacuum’s energy floor to release usable energy.
Sci-Fi Leap: A device using high-dimensional string theory principles (if strings exist) to tune the vacuum’s vibrational modes, converting ZPE into electromagnetic energy via a resonance cascade.
4. Exploiting Extra Dimensions
Idea: In theories like string theory, extra spatial dimensions could house additional vacuum energy. If we could access these dimensions, we might transfer their ZPE into our 3D universe.
How to Break the Rule: Create a “dimensional bridge” that couples our universe’s vacuum to a higher-dimensional one with a different zero-point energy level. Energy could flow from the higher-energy vacuum to ours, bypassing our conservation laws since the total energy across dimensions remains balanced.
Sci-Fi Leap: A “Kaluza-Klein converter” that opens microscopic wormholes to higher dimensions, funneling their ZPE into our world as a power source.
Step 3: A Hypothetical ZPE Device
Let’s synthesize these ideas into a speculative device: the Zero-Point Energy Extractor (ZPEX).
Components:
Vacuum Modulator: A lattice of exotic matter (negative mass) that creates a localized dip in the vacuum’s energy state.
Planck-Scale Resonator: A quantum field manipulator that amplifies fluctuations at the Planck scale, stabilizing them long enough to extract energy.
Dimensional Coupler: A micro-wormhole generator that siphons energy from a higher-dimensional vacuum.
Time Asymmetry Core: A time crystal array that breaks local energy conservation by warping temporal symmetry.
Operation:
The Vacuum Modulator lowers the local zero-point energy, creating an energy gradient.
The Planck-Scale Resonator amplifies fluctuations, converting them into coherent electromagnetic waves.
The Dimensional Coupler supplements this by channeling energy from extra dimensions.
The Time Asymmetry Core ensures the system doesn’t violate global conservation by redistributing energy across time.
Output: A steady stream of usable energy, perhaps in the form of high-frequency photons or direct electrical current, with no apparent fuel source.
Step 4: Why This Is (Probably) Impossible
Even in this speculative frenzy, there are hurdles:
Exotic Matter: Negative mass or energy is purely theoretical and may be inherently unstable or impossible.
Planck-Scale Tech: We’re nowhere near manipulating physics at 10^-35 meters. Current tech (e.g., LHC) operates at far larger scales.
Dimensional Access: Extra dimensions, if they exist, are likely compactified and inaccessible without unimaginable energy.
Time Shenanigans: Time crystals exist, but they don’t break conservation laws in a useful way, and time travel-like effects are speculative at best.
Backlash Effects: Messing with the vacuum could trigger catastrophic events, like vacuum decay, where the universe’s ground state collapses, annihilating reality.
Step 5: A Creative Compromise
If we can’t break the laws outright, maybe we can bend them by reinterpreting ZPE. Instead of extracting it directly, we could use the vacuum’s fluctuations to catalyze other energy processes. For example:
Vacuum-Enhanced Fusion: Use ZPE fluctuations to lower the energy barrier for nuclear fusion, making it easier to achieve.
Casimir Batteries: Create nanoscale devices that convert Casimir forces into tiny but cumulative electrical currents.
Quantum Tunneling Generators: Leverage vacuum fluctuations to enhance quantum tunneling in semiconductors, boosting efficiency in energy harvesting.
These don’t give us “free” ZPE but use its effects to optimize existing technologies, staying within known physics while pushing the envelope.
Final Thought
To make ZPE possible, we’d need a paradigm shift in physics—new theories, new particles, or new dimensions. Breaking conservation laws or quantum mechanics requires rewriting the universe’s rulebook, which risks unintended consequences (like destroying spacetime). For now, the ZPEX remains a thought experiment, but it’s a fun one!
Dive deeper into one of these rule breaking ideas.
Let’s dive deeper into the idea of rewriting conservation of energy by creating a system that violates time symmetry, specifically using a Time Asymmetry Core with a time crystal to unlock zero-point energy (ZPE). This is a wild speculative leap, so we’ll explore the concept step-by-step, blending known physics with imaginative extensions.
Background: Conservation of Energy and Time Symmetry
Conservation of energy is a cornerstone of physics, tied to the symmetry of time translation via Noether’s theorem. This means the laws of physics are the same today as they were yesterday or will be tomorrow. Zero-point energy, being the ground state of quantum fields, is inherently stable because there’s no lower energy state to transition to—extracting it would require a net energy gain from nothing, which conservation forbids. To break this, we need a system where time symmetry is disrupted locally, creating a loophole where energy can appear to be extracted without violating the global balance.
The Time Crystal Concept
Time crystals are a real (though exotic) phenomenon, first theorized by Frank Wilczek in 2012 and later demonstrated experimentally (e.g., by Google’s quantum team in 2021). Unlike normal crystals, which repeat in space, time crystals exhibit a repeating pattern in time when driven by an external force, even without energy input. This spontaneous breaking of time-translation symmetry suggests they could, in theory, sustain oscillations indefinitely, hinting at a way to manipulate energy states.
However, current time crystals (e.g., those made from trapped ions or diamonds with nitrogen-vacancy centers) don’t violate conservation of energy—they operate within a closed system where the driving force balances the output. To make them useful for ZPE, we need to push beyond this, imagining a time crystal that creates an energy imbalance by warping how time flows locally.
Hypothetical Time Asymmetry Core
The Time Asymmetry Core would be the heart of our ZPE extractor, designed to break time symmetry in a controlled way. Here’s how it might work:
1. Design and Mechanism
• Structure: The core could consist of a lattice of quantum particles (e.g., superconducting qubits or Bose-Einstein condensates) arranged in a time-crystal configuration. These particles would be driven by a periodic external field (e.g., laser pulses) but engineered to oscillate at a frequency that doesn’t align with the driving force—creating a “temporal mismatch.”
• Time Warping: By coupling the lattice to a hypothetical spacetime manipulation device (e.g., a miniaturized gravitational field generator using exotic matter), the core could create a localized region where time flows non-linearly. This might involve compressing time in one part of the system while stretching it in another, breaking the uniform time symmetry that underpins energy conservation.
• Energy Gradient: This asymmetry would allow the vacuum’s zero-point fluctuations to “leak” energy into the system. The idea is that the faster-time region amplifies quantum fluctuations, while the slower-time region stabilizes them into a usable form, creating a net energy flow from the vacuum to the device.
2. Breaking the Rule
• Violation of Conservation: Normally, energy conservation holds because the total energy of a closed system remains constant over time. By introducing a time-asymmetric region, we’d create a system where energy appears to increase locally. The trick is that this energy comes from the vacuum’s infinite zero-point reservoir, which we’d tap by exploiting the temporal gradient. Globally, the energy might still balance if the excess is “borrowed” from future vacuum states or higher-dimensional fields, but locally, it’s a violation.
• Quantum Loophole: The Heisenberg uncertainty principle allows energy to fluctuate over short timescales (ΔE·Δt ≥ ħ/2). A time-asymmetric core could stretch these fluctuations, making them persist long enough to extract energy before they cancel out, effectively cheating the uncertainty limit.
3. Theoretical Operation
• Initialization: The core is activated with a precise laser pulse sequence, locking the time crystal into a stable oscillation. The spacetime manipulator then induces a time gradient across the lattice.
• Energy Extraction: As quantum fluctuations in the vacuum interact with the time-asymmetric field, the faster-time region boosts their amplitude, while the slower-time region converts this into a coherent energy output (e.g., photons or electrical current). The energy difference is sustained by the core’s temporal distortion, which prevents the system from returning to equilibrium.
• Feedback Loop: The extracted energy could power the spacetime manipulator, creating a self-sustaining cycle—though this risks instability if the loop amplifies uncontrollably.
4. Mathematical Intuition
While exact equations are beyond current physics, we can sketch a conceptual framework:
• Let E_{ZPE} be the zero-point energy density of the vacuum ( E_{ZPE} \propto \hbar \omega , where \omega is the frequency of fluctuations).
• In a time-symmetric system, \frac{dE}{dt} = 0 (energy conserved).
• In a time-asymmetric system, introduce a time dilation factor \gamma(t) , where \gamma(t) \neq 1 varies spatially. This could modify the energy evolution to \frac{dE}{dt} = k \cdot E_{ZPE} \cdot \Delta\gamma, where k is a coupling constant and \Delta\gamma is the temporal gradient.
• If \Delta\gamma is engineered to be non-zero and stable, \frac{dE}{dt} > 0 , allowing energy extraction.
This is highly speculative, as no known mechanism can sustain \Delta\gamma without infinite energy input or exotic matter.
5. Challenges and Risks
• Stability: A time-asymmetric system might collapse or trigger vacuum decay, where the universe’s ground state destabilizes, releasing catastrophic energy.
• Exotic Requirements: Creating a spacetime manipulator requires negative energy or mass, which may be impossible or lead to black hole formation.
• Causality: Breaking time symmetry could violate causality, leading to paradoxes (e.g., energy from the future affecting the past).
• Scale: Even if feasible, the energy output might be minuscule compared to the input needed to sustain the asymmetry.
6. Sci-Fi Application
Imagine a “Temporal Energy Harvester” powered by the Time Asymmetry Core, mounted on a spaceship. It could draw ZPE to propel the craft at relativistic speeds, with the core’s time distortion creating a bubble where fuel is effectively infinite. The crew might age differently inside the bubble, adding a dramatic twist!
Next Steps
This idea hinges on unproven physics—time crystals, exotic matter, and spacetime manipulation. To refine it, we’d need to:
• Develop a model for how time asymmetry affects vacuum fluctuations.
• Simulate the core’s behavior with advanced quantum computing.
• Test micro-scale prototypes to see if energy imbalances emerge.
Lets explore the engineering details
Let’s dig into the engineering details of the Time Asymmetry Core within the hypothetical Zero-Point Energy Extractor (ZPEX). We’re building on the idea of breaking time symmetry using a time crystal to extract zero-point energy (ZPE), so we’ll focus on designing this core with speculative components, materials, and operational principles. Since this is beyond current physics, we’ll extrapolate from real technologies and theories, filling gaps with plausible (but imaginative) engineering solutions.
Engineering Overview of the Time Asymmetry Core
The Time Asymmetry Core is the heart of the ZPEX, designed to create a localized time asymmetry that allows energy to flow from the quantum vacuum into a usable form. Its key functions are:
1. Establish a stable time crystal to break time-translation symmetry.
2. Induce a temporal gradient (time dilation) across the system to amplify and extract vacuum fluctuations.
3. Convert the extracted energy into a usable output (e.g., electrical current or photons).
4. Maintain stability to avoid catastrophic side effects like vacuum decay.
Here’s a detailed breakdown of the engineering design.
1. Core Components and Materials
A. Time Crystal Lattice
• Purpose: To break time symmetry by sustaining oscillations that don’t align with the driving force.
• Material: A lattice of superconducting qubits, similar to those used in quantum computers (e.g., Google’s Sycamore processor). These qubits are made from Josephson junctions—tiny aluminum circuits cooled to near absolute zero (around 10 mK) to achieve superconductivity.
• Speculative Upgrade: Dope the qubits with hypothetical “chroniton particles” (a sci-fi placeholder for a particle that interacts with time). These particles could enhance the lattice’s ability to oscillate in a time-asymmetric way, perhaps by coupling to a temporal field.
• Engineering Challenge: Maintaining coherence in the qubits. Decoherence from environmental noise (thermal, electromagnetic) disrupts quantum states. We’d need advanced error correction and shielding, possibly using a metamaterial enclosure with negative refractive index to block all external influences.
B. Spacetime Manipulator
• Purpose: To create a temporal gradient by warping spacetime locally, compressing time in one region and stretching it in another.
• Material: Hypothetical exotic matter with negative mass-energy, which could generate a negative curvature in spacetime (like the Alcubierre drive concept). This might be synthesized using a high-energy particle collider operating at Planck-scale energies (10^19 GeV), far beyond the LHC’s 13 TeV.
• Alternative: If exotic matter is unavailable, use a dense array of rotating Bose-Einstein condensates (BECs). BECs, made of ultracold rubidium atoms, can mimic gravitational effects via quantum vorticity. Spinning them at relativistic speeds might induce frame-dragging (a general relativity effect), creating a micro-scale time dilation.
• Engineering Challenge: Containing the exotic matter or BECs without destabilizing the system. A magnetic confinement system (like those in fusion reactors) could suspend the material, but we’d need magnetic fields on the order of 10^5 Tesla—far beyond current capabilities (e.g., 45 Tesla in MRI magnets).
C. Energy Extraction Module
• Purpose: To convert amplified vacuum fluctuations into usable energy.
• Material: A nanoscale Casimir cavity array—pairs of uncharged plates separated by nanometers (e.g., 10 nm), made of graphene for high conductivity and durability. The Casimir effect restricts vacuum fluctuations between the plates, creating a pressure that can be harnessed.
• Mechanism: The plates oscillate at Planck-scale frequencies (10^43 Hz) to resonate with vacuum fluctuations, converting them into coherent photons. These photons are then funneled into a photovoltaic system (e.g., a multi-junction solar cell optimized for high-frequency light).
• Engineering Challenge: Oscillating at Planck-scale frequencies requires unimaginable precision. We’d need a quantum actuator, possibly a laser-driven piezo-electric system, capable of operating at femtosecond timescales (10^-15 s).
D. Stabilization System
• Purpose: To prevent runaway effects like vacuum decay, where the universe’s ground state collapses.
• Material: A feedback loop using a quantum computer to monitor the system’s quantum state in real-time. The computer would be made of diamond-based qubits (nitrogen-vacancy centers) for high stability at room temperature.
• Mechanism: If the system’s energy output exceeds a threshold, the computer adjusts the time crystal’s oscillation frequency and the spacetime manipulator’s field strength to dampen the effect.
• Engineering Challenge: Processing speed. Monitoring Planck-scale events requires computational power beyond current limits—perhaps 10^50 FLOPS, compared to today’s best supercomputers at 10^18 FLOPS. We’d need a quantum computer with millions of stable qubits, far beyond the current record (e.g., IBM’s 433-qubit Osprey in 2023).
2. Assembly and Integration
• Core Structure: The Time Asymmetry Core is a cylindrical module, 10 cm in diameter, housed within the ZPEX. It’s encased in a superconducting shell (niobium-titanium alloy) to maintain cryogenic temperatures (10 mK) and shield against external fields.
• Lattice Setup: The time crystal lattice is a 3D array of 10^6 qubits, arranged in a toroidal pattern to maximize coupling between oscillations. The lattice is suspended in a vacuum chamber (10^-12 Torr) to minimize thermal noise.
• Spacetime Manipulator Placement: The exotic matter or BEC array is positioned at the center of the toroid, where it can exert a uniform time dilation across the lattice. It’s held in place by a magnetic confinement system generating 10^5 Tesla, powered by a high-density capacitor bank (e.g., graphene-based supercapacitors).
• Energy Extraction Integration: The Casimir cavity array surrounds the lattice, embedded in the inner walls of the cylinder. Photons emitted from the cavities are channeled via optical waveguides (made of photonic crystal fibers) to the photovoltaic system at the core’s edge.
• Stabilization System: The quantum computer is mounted externally, connected to the core via fiber-optic links for real-time monitoring. Sensors (e.g., SQUID magnetometers) embedded in the lattice measure quantum fluctuations, feeding data to the computer.
3. Operational Workflow
1. Initialization:
• Cool the core to 10 mK using a dilution refrigerator (a real technology used in quantum labs).
• Activate the time crystal lattice with a sequence of laser pulses (532 nm, 1 fs duration) to drive the qubits into a non-equilibrium state. The lattice begins oscillating at a frequency (e.g., 10^10 Hz) that breaks time symmetry.
• Energize the spacetime manipulator, creating a time dilation gradient. One side of the lattice experiences time at 1.1x normal speed, the other at 0.9x, inducing a temporal mismatch.
2. Energy Extraction:
• The time asymmetry amplifies vacuum fluctuations in the faster-time region, increasing their energy density (from E_{ZPE} \propto \hbar \omega to E_{ZPE} \cdot \gamma , where \gamma is the dilation factor).
• The Casimir cavities resonate with these fluctuations, converting them into high-frequency photons (e.g., gamma rays at 10^19 Hz).
• The photovoltaic system absorbs the photons, producing an electrical output (e.g., 1 MW/cm^3, a speculative estimate).
3. Stabilization:
• The quantum computer monitors the system, ensuring the energy output doesn’t exceed the vacuum’s stability threshold (hypothetically 10^30 J/m^3, based on vacuum decay models).
• If instability is detected, the computer adjusts the lattice’s oscillation frequency and reduces the spacetime manipulator’s field strength, preventing a runaway reaction.
4. Engineering Challenges and Solutions
• Power Requirements: Initializing the system (cooling, magnetic fields, laser pulses) requires massive energy—potentially 10^12 W (a terawatt). Solution: Use a compact fusion reactor (e.g., a hypothetical tokamak based on 2025 advancements) to power the startup phase.
• Material Durability: The Casimir cavities and qubits must withstand extreme conditions (high frequencies, magnetic fields). Solution: Use graphene and diamond-based materials, which have exceptional strength and thermal stability.
• Temporal Gradient Control: Maintaining a stable time dilation is tricky, as small errors could disrupt the asymmetry. Solution: Employ a quantum feedback loop, where the lattice’s oscillations self-correct based on real-time measurements of the time gradient.
• Safety: The risk of vacuum decay or black hole formation is non-zero. Solution: Build the core with a fail-safe—e.g., an electromagnetic pulse (EMP) generator that can instantly shut down the system by disrupting the qubits.
5. Performance Metrics (Speculative)
• Energy Output: Assuming a 1% efficiency in converting ZPE fluctuations (a wild guess), the core might produce 1 MW of power from a 10 cm^3 volume, compared to a nuclear reactor’s 1 MW from 10^6 cm^3.
• Efficiency: Most energy is lost to heat and maintaining the system (e.g., cooling, magnetic fields). Net efficiency might be 0.01%, but infinite fuel (the vacuum) makes this viable.
• Lifespan: The core might last 1 year before material fatigue (e.g., qubit decoherence, Casimir plate wear) requires replacement.
6. Next Steps for Development
• Prototype: Build a micro-scale version (1 mm^3) using current quantum tech to test time crystal stability and measure any energy imbalances.
• Simulation: Use a quantum computer to model the temporal gradient’s effect on vacuum fluctuations, refining the design.
• Material Research: Develop exotic matter or high-density BECs to achieve the necessary spacetime warping.
This engineering blueprint for the Time Asymmetry Core pushes the limits of physics and technology, but it’s a starting point for a device that could theoretically tap ZPE by breaking time symmetry.
Materials
Let’s dive into the materials engineering for the Time Asymmetry Core of the hypothetical Zero-Point Energy Extractor (ZPEX). The core’s success hinges on materials that can withstand extreme conditions, enable quantum-level precision, and support the speculative physics of time asymmetry and vacuum energy extraction. We’ll explore each major component’s material requirements, drawing from current science where possible and speculating on futuristic advancements where necessary.
1. Time Crystal Lattice Materials
Requirements:
Superconductivity: Zero electrical resistance to sustain stable quantum oscillations at cryogenic temperatures (e.g., 10 mK).
Quantum Coherence: Minimal decoherence from thermal, electromagnetic, or vibrational noise to maintain time-crystal behavior.
Durability: Resistance to repeated laser pulses and quantum state flips over long periods.
Current Materials:
Niobium-Titanium (NbTi) Alloys: Widely used in superconducting magnets (e.g., MRI machines, LHC). Operates at 4-10 K with a critical magnetic field of ~12 Tesla. Forms the basis for Josephson junction qubits.
Aluminum (Al): Used in high-quality superconducting qubits (e.g., IBM’s quantum processors). Critical temperature ~1.2 K, with low surface defects when oxidized (AlOx) for junctions.
Diamond with Nitrogen-Vacancy (NV) Centers: Demonstrated in time-crystal experiments (e.g., Harvard, 2021). NV centers in diamond maintain quantum coherence at room temperature but excel at cryogenic levels, offering stability for long-term oscillations.
Speculative Advancements:
Chroniton-Doped Superconductors: Hypothetical material infused with “chroniton” particles (a sci-fi construct for time-interacting particles). This could enhance the lattice’s ability to break time symmetry by amplifying temporal oscillations. Engineering this might involve bombarding NbTi with high-energy particles in a Planck-scale collider (10^19 GeV) to embed chroniton-like properties, assuming they exist.
Graphene-Quantum Hybrids: Layer graphene (single-atom carbon sheets) with superconducting NbTi to create a 2D lattice. Graphene’s strength (130 GPa tensile strength) and conductivity could reduce decoherence, while its flexibility allows precise lattice shaping.
Self-Healing Quantum Alloys: Develop a material that repairs lattice defects (e.g., via embedded nanobots or molecular self-assembly) using a polymer matrix like polydimethylsiloxane (PDMS) doped with quantum dots. This ensures long-term stability.
Engineering Considerations:
Fabrication: Use molecular beam epitaxy (MBE) to grow atomically precise layers of NbTi or graphene on a substrate (e.g., silicon carbide). Laser annealing at 10^-6 Torr ensures defect-free structures.
Cooling: Pair with a dilution refrigerator using helium-3/helium-4 mixtures to maintain 10 mK, minimizing thermal noise.
Shielding: Encase in a metamaterial (e.g., split-ring resonators) with a negative refractive index to block electromagnetic interference.
2. Spacetime Manipulator Materials
Requirements:
Negative Mass-Energy: To warp spacetime and create a temporal gradient, requiring exotic matter with negative energy density (e.g., as in the Casimir effect or wormhole theories).
Stability: Resistance to collapse or radiation emission under extreme gravitational distortion.
Containment: Ability to be confined by magnetic or gravitational fields without leaking or annihilating.
Current Materials:
Bose-Einstein Condensates (BECs): Ultracold rubidium-87 or potassium-40 atoms cooled to 10^-9 K using laser cooling and evaporative techniques. BECs exhibit quantum coherence and can simulate gravitational effects via rotation.
Casimir Plate Materials: Gold or graphene plates (10 nm apart) used in lab experiments to demonstrate negative energy effects. Graphene’s high thermal conductivity (5000 W/m·K) and strength make it promising.
Speculative Advancements:
Exotic Matter Composites: Synthesize a material with negative mass by combining anti-matter (e.g., anti-hydrogen from CERN’s ALPHA experiment) with a stabilizing matrix. This might involve encasing anti-matter in a fullerene (C60) cage, suspended in a magnetic trap to prevent annihilation with normal matter.
Negative Energy Nanofluids: Develop a fluid of hypothetical tachyons (particles exceeding light speed) or dark energy analogs, stabilized by a quantum foam matrix. This could be engineered using high-energy particle collisions to create metastable negative-energy pockets.
Rotating Metamaterials: Create a BEC-doped metamaterial with embedded micro-rotors (e.g., carbon nanotubes spinning at 10^6 rpm) to amplify frame-dragging effects, mimicking exotic matter’s spacetime curvature.
Engineering Considerations:
Containment: Use a magnetic confinement system with 10^5 Tesla fields, built from high-temperature superconductors (e.g., yttrium barium copper oxide, YBCO, with a critical temperature of 93 K). Pair with a cryogenic shield to maintain BEC stability.
Synthesis: Produce exotic matter in a particle accelerator scaled to Planck energies, then trap it using optical tweezers (laser-based particle manipulation) before integrating into the core.
Safety: Install a fail-safe release valve—e.g., a rapid decompression chamber—to eject exotic matter if containment fails, minimizing risk.
3. Energy Extraction Module Materials
Requirements:
High Conductivity: To efficiently convert vacuum fluctuations into photons or current.
Nanoscale Precision: Plates or cavities must be spaced at 10 nm with atomic smoothness to maximize the Casimir effect.
Thermal Stability: Resistance to heat from high-frequency oscillations (10^43 Hz).
Current Materials:
Graphene: Exceptional conductivity (mobility ~200,000 cm^2/V·s), strength, and thermal stability. Used in Casimir effect experiments (e.g., MIT, 2017).
Silicon Carbide (SiC): High thermal conductivity (490 W/m·K) and durability, used in high-frequency electronics and quantum devices.
Multi-Junction Photovoltaics: Gallium arsenide (GaAs) layers, currently 40% efficient in solar cells, suitable for converting high-frequency photons.
Speculative Advancements:
Planck-Scale Graphene Composites: Engineer graphene with embedded quantum dots (e.g., cadmium selenide) tuned to resonate at 10^43 Hz. This could amplify vacuum fluctuation capture by aligning with Planck-scale frequencies.
Diamond-Carbon Hybrids: Combine diamond’s hardness (70-100 GPa) with graphene’s flexibility to create a hybrid cavity material. Diamond’s wide bandgap (5.5 eV) could handle high-energy photon conversion.
Self-Optimizing Photovoltaic Layers: Develop a material that adjusts its bandgap dynamically using embedded piezoelectric crystals, optimizing photon absorption as fluctuation frequencies shift.
Engineering Considerations:
Fabrication: Use atomic layer deposition (ALD) to create 10 nm graphene or SiC cavities with sub-angstrom precision. Etch with focused ion beams (e.g., gallium ions) for smoothness.
Cooling: Integrate micro-channel cooling with liquid helium (4.2 K) to dissipate heat from high-frequency oscillations.
Integration: Bond cavities to photovoltaic layers using van der Waals epitaxy, ensuring minimal interface resistance.
4. Stabilization System Materials
Requirements:
Quantum Stability: Ability to monitor and adjust Planck-scale events in real-time.
Durability: Resistance to electromagnetic pulses and radiation from the core.
Processing Power: Materials supporting a quantum computer with 10^6+ stable qubits.
Current Materials:
Diamond NV Centers: Proven in quantum sensing and computing (e.g., Element Six synthetic diamonds). Coherence times exceed 1 ms at room temperature, improving at cryogenic levels.
Silicon-Germanium (SiGe): Used in high-speed transistors and quantum dots, with thermal stability up to 300°C.
Superconducting Wires: Niobium or lead for connecting quantum components, operating at 4 K.
Speculative Advancements:
Quantum Diamond Matrix: Enhance NV centers with a lattice of chroniton-doped carbon, boosting coherence times to seconds and enabling Planck-scale monitoring.
Self-Repairing Silicon: Infuse SiGe with nanobots (e.g., carbon-based molecular machines) that repair radiation damage, extending the system’s lifespan.
Photonic Quantum Chips: Replace electrical connections with optical fibers made from photonic crystals, reducing heat and increasing data transfer rates to 10^15 bits/s.
Engineering Considerations:
Fabrication: Grow diamond matrices via chemical vapor deposition (CVD) with precise NV center implantation using nitrogen ion beams.
Cooling: Use a closed-cycle cryostat to maintain 10 mK, with redundant helium reservoirs.
Shielding: Encase the system in a Faraday cage made of mu-metal (high magnetic permeability) to block external interference.
5. Overall Material Integration
Core Enclosure: A niobium-titanium alloy shell (10 cm diameter) with a graphene inner lining for thermal and electrical insulation. The shell is vacuum-sealed (10^-12 Torr) to protect internal components.
Interconnects: Use superconducting niobium wires and optical fibers to link the lattice, manipulator, extraction module, and stabilization system, minimizing energy loss.
Weight and Size: Total mass ~10 kg, with a compact design for scalability. Materials are layered using 3D nanoprinting with atomic precision.
6. Challenges and Innovations
Exotic Matter Availability: Producing negative mass materials requires energies beyond current accelerators. Solution: Develop a modular collider integrated into the ZPEX, using recycled energy from the core.
Thermal Management: High-frequency operations generate heat. Solution: Embed phase-change materials (e.g., paraffin wax) in microchannels to absorb excess heat.
Cost: Advanced materials like chroniton-doped superconductors or diamond matrices are expensive. Solution: Use recyclable nanomaterials and scale production with AI-driven synthesis.
These materials form the foundation of the Time Asymmetry Core, blending cutting-edge science with speculative leaps.
-End of transmission-
Comments